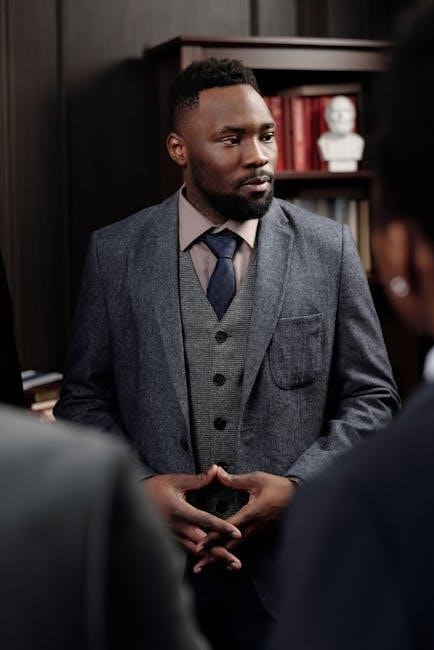
Thermodynamics is the scientific study of work, heat, and energy transfer. It examines the fundamental laws governing energy transformations, providing insights into system behavior and efficiency across various fields.
1.1 Definition and Scope of Thermodynamics
Thermodynamics is the scientific study of energy, work, and heat transfer, focusing on the physical properties of systems in equilibrium. It explores how energy transforms between forms and interacts with matter. The scope of thermodynamics encompasses both theoretical and practical applications, addressing phenomena at macroscopic scales. It provides fundamental principles for understanding efficiency, entropy, and energy conservation, applicable across engineering, physics, chemistry, and environmental sciences. Thermodynamics is rooted in four core laws, which govern all energy-related processes.
1.2 Historical Development of Thermodynamics
Thermodynamics emerged in the 19th century, driven by the study of heat engines and energy conversion. Sadi Carnot’s work on engine efficiency laid the foundation, while Rudolf Clausius introduced the concept of entropy. William Thomson (Lord Kelvin) contributed to the absolute temperature scale. These pioneers, along with others, established the laws of thermodynamics, blending theoretical insights with experimental evidence. Their work revolutionized physics, engineering, and chemistry, shaping our understanding of energy and its transformations. This historical journey underscores thermodynamics’ enduring relevance in science and technology.
The Zeroth Law of Thermodynamics
The Zeroth Law establishes the concept of thermal equilibrium, enabling the definition of temperature. It states that if two systems are in equilibrium with a third, they are also in equilibrium with each other, forming the basis for temperature measurement and thermodynamic systems.
2.1 Thermal Equilibrium and the Concept of Temperature
Thermal equilibrium occurs when a system ceases to exchange heat with its surroundings or other systems. This state is fundamental to thermodynamics, as it allows for the definition of temperature, a measurable property indicating the thermal state of a system. The Zeroth Law of Thermodynamics formalizes this concept, enabling the creation of temperature scales and ensuring consistency in thermal measurements. Temperature serves as a critical parameter in understanding and predicting the behavior of thermodynamic systems, making it indispensable in scientific and engineering applications.
2.2 The Role of Thermometers in Thermodynamics
Thermometers play a vital role in thermodynamics by measuring temperature, a fundamental property of systems in thermal equilibrium. They enable the quantification of heat transfer and energy exchange, which are central to thermodynamic processes; By providing precise temperature readings, thermometers allow for the validation of theoretical models and the application of thermodynamic laws. Their accuracy ensures reliable data collection, making them indispensable tools in both scientific research and practical engineering applications. Thermometers bridge theory and experimentation, facilitating deeper insights into energy behavior and system interactions.
The First Law of Thermodynamics
The First Law of Thermodynamics states that energy cannot be created or destroyed in an isolated system, only transformed from one form to another, ensuring conservation.
3.1 The Conservation of Energy Principle
The conservation of energy principle is a fundamental concept in thermodynamics, stating that energy cannot be created or destroyed, only transformed from one form to another. This principle applies universally to all physical processes, ensuring that the total energy of an isolated system remains constant. It forms the basis of the First Law of Thermodynamics, emphasizing that energy is conserved in every interaction, whether as heat, work, or other forms. This principle is crucial in understanding the balance of energy in systems across physics, engineering, and natural sciences, providing a foundation for calculating energy changes and system behavior.
3.2 Mathematical Formulation: ΔU = Q — W
The first law of thermodynamics is mathematically expressed as ΔU = Q — W, where ΔU represents the change in internal energy of a system. Q is the heat added to the system, and W is the work done by the system. This equation signifies that the net energy change in a closed system is the difference between the heat supplied and the work performed. It embodies the principle of energy conservation, ensuring that energy is neither created nor destroyed, only transformed between forms. This formulation is foundational for analyzing thermodynamic processes and calculating energy balances in various systems.
The Second Law of Thermodynamics
The second law introduces the concept of entropy, a measure of disorder, and explains the unidirectional nature of natural processes. It governs energy transformations, ensuring efficiency limits and defining the arrow of time, while prohibiting perpetual motion machines of the second kind. This law is fundamental to understanding irreversible processes and the degradation of energy quality in systems.
4.1 Entropy and the Arrow of Time
Entropy, a measure of disorder, lies at the core of the second law. It explains why natural processes progress in one direction, establishing the “arrow of time.” As entropy increases, systems tend toward disorder, making irreversible processes inherent in the universe. The second law quantifies this through the formula ΔS = Q_rev/T, where S is entropy, Q_rev is reversible heat transfer, and T is temperature. This principle underscores the inevitability of energy degradation and the unidirectional nature of time in thermodynamic systems.
4.2 Irreversible Processes and the Efficiency of Energy Conversion
Irreversible processes, such as heat transfer and friction, inherently reduce the efficiency of energy conversion. The second law introduces the concept of entropy increase, which limits the maximum efficiency of any system. No process can achieve 100% efficiency, as some energy is always lost to the environment. This principle underscores the practical limitations of energy transformation, emphasizing that real-world systems cannot operate without entropy generation, thus setting bounds on technological advancements and energy utilization.
The Third Law of Thermodynamics
The Third Law of Thermodynamics defines the behavior of systems near absolute zero. It states that entropy approaches zero for perfect crystals, guiding low-temperature physics and material science.
5.1 The Concept of Absolute Zero
Absolute zero is the theoretical temperature at which particles possess minimal thermal motion, defined as 0 Kelvin, -273.15°C, or -459.67°F. It represents the lower bound of temperature, where entropy approaches zero for perfect crystals. Achieving absolute zero is impossible due to quantum effects and the Third Law of Thermodynamics, which states that infinite steps would be required to remove all entropy. This concept underpins low-temperature physics and the behavior of matter at its coldest extremes, guiding advancements in cryogenics and material science.
5.2 Implications for Perfect Crystals and Entropy
A perfect crystal at absolute zero possesses minimal entropy, representing a state of maximum order. The Third Law of Thermodynamics implies that as a system approaches absolute zero, its entropy approaches a minimum value, often zero for ideal crystals. This principle highlights the interplay between temperature, order, and energy distribution. The concept of perfect crystals and their entropy provides foundational insights into material behavior at extreme temperatures, influencing fields like cryogenics and quantum physics.
Applications of the Laws of Thermodynamics
Thermodynamics’ principles are vital in engineering, environmental science, and material research, governing energy efficiency, system design, and understanding natural processes across scales.
6.1 Engineering and Technological Applications
The laws of thermodynamics are foundational in engineering, guiding the design of power plants, refrigeration systems, and engines. They optimize energy conversion, ensuring efficiency in heat transfer and work output. The first law, focusing on energy conservation, is crucial for analyzing systems like internal combustion engines. The second law, introducing entropy, helps evaluate the maximum efficiency of heat pumps and turbines. These principles are essential for advancing technologies, enabling engineers to develop sustainable and high-performance solutions across industries.
6.2 Environmental and Natural Science Implications
Thermodynamics provides critical insights into environmental and natural systems. The second law, through entropy, explains the direction of natural processes, such as heat transfer and energy degradation. This is vital for understanding climate change, where entropy increases as energy disperses. It also informs ecological balance, linking energy flow to ecosystem health. Thermodynamics aids in assessing the efficiency of natural resource use and predicting the environmental impact of industrial processes, guiding sustainable practices and resource management to mitigate ecological degradation and promote energy conservation.
Key Figures in the Development of Thermodynamics
Pioneers like Sadi Carnot, Rudolf Clausius, and William Thomson laid the groundwork for thermodynamics. Their work established foundational principles, shaping our understanding of energy and its transformations.
7.1 Sadi Carnot and the Foundations of the Second Law
Sadi Carnot, a French physicist, is renowned for his groundbreaking work in thermodynamics. His 1824 book, Reflections on the Motive Power of Fire, laid the theoretical foundation for the second law. Carnot introduced the concept of the heat engine, analyzing the efficiency of energy conversion. He proposed that the maximum efficiency of any heat engine is determined by the temperature difference between the hot and cold reservoirs. Carnot’s ideas, though initially theoretical, influenced later scientists like Rudolf Clausius, who formulated the second law. His work remains a cornerstone of thermodynamics, emphasizing the direction of energy transformations and the limitations of energy conversion.
7.2 Rudolf Clausius and the Concept of Entropy
Rudolf Clausius, a German physicist, significantly advanced thermodynamics by introducing the concept of entropy in 1865. He defined entropy as a measure of energy dispersal, linking it to the second law. Clausius formulated the mathematical expression for entropy change, ΔS = dQ/T, and emphasized its role in irreversible processes. His work established entropy as a fundamental property, revealing its connection to the arrow of time. Clausius’s contributions remain foundational, shaping modern understanding of energy transformations and system behavior in thermodynamics.
Thermodynamics in Modern Contexts
Thermodynamics in modern contexts explores quantum systems and biological processes, advancing our understanding of energy principles in cutting-edge scientific and technological applications, enhancing sustainability.
8.1 Quantum Thermodynamics and New Frontiers
Quantum thermodynamics explores the intersection of thermodynamic principles with quantum mechanics, revealing new insights into energy and entropy at microscopic scales. Recent studies examine how thermodynamic laws apply to quantum systems, such as energy fluctuations and entropy production in small-scale processes. These advancements challenge classical assumptions and open doors to innovative technologies, including quantum engines and refrigerators. The field also investigates the thermodynamics of information, linking concepts like Maxwell’s demon to quantum information theory. This emerging area bridges fundamental physics with cutting-edge technological applications, reshaping our understanding of energy and its limits in quantum realms.
8.2 Biological Thermodynamics and Living Systems
Biological thermodynamics applies thermodynamic principles to living systems, exploring how organisms manage energy and entropy. Living systems maintain order by exchanging energy with their environment, aligning with the second law of thermodynamics. Metabolic processes, such as photosynthesis and respiration, exemplify energy conversion and efficiency. Thermodynamic analysis also aids in understanding disease mechanisms and aging, where energy imbalances occur. This field bridges biology and physics, offering insights into life’s sustainability and ecological systems’ functioning.
Leave a Reply
You must be logged in to post a comment.